Van Andel Institute’s Dr. Huilin Li is using modern technology to solve an ancient problem that still has devastating consequences today: tuberculosis (TB), a disease that has plagued humanity throughout known history.
Called phthisis by the ancient Greeks, tabes by the ancient Romans and schachepheth by the ancient Hebrews, tuberculosis didn’t receive its modern moniker until 1834.1 Even after, it still was known by its then-common name, consumption, a reflection of the disease’s ability to wholly “consume” a person’s health and wellbeing.2 Its most common symptoms are chronic cough, weakness, fatigue and weight loss. 3 Although preventable and curable, TB can be deadly if left untreated.3
For much of human history, the cause of TB was unknown. Then, on March 24, 1882, Dr. Robert Koch announced he had identified Mycobacterium tuberculosis as the bacteria behind the disease.1 This breakthrough fueled more than a century of research and discovery that has saved countless lives.
Despite our best efforts, however, tuberculosis remains a threat today.
Li and his colleagues are working to change that. Thanks to rapid advances in technique and technology, they have powerful tools at their disposal, including high-powered microscopes that are helping chip away at TB’s defenses.
“Tuberculosis is a very old disease with a wealth of survival strategies in its playbook,” Li says. “But with all that we know — and all that we’re learning — we have a few tricks up our sleeves, too.”
New solutions to an old problem
At first glance, Van Andel Institute’s largest cryo-electron microscope might be confused with an industrial refrigerator. Ten feet tall, gray and black, and unassuming — without context, few people would realize they were looking at one of the most powerful microscopes in the world. But a peek inside the machine reveals a marvel of engineering that enables scientists to see some of life’s smallest building blocks.
Unlike standard microscopes, which use glass lenses to magnify materials mounted on slides, cryo-electron microscopes work by beaming electrons through a frozen sample. The resulting “shadows” cast by the sample, usually a protein or other molecule, are collected by the cryo-EM’s state-of-the-art software and collated into 3D images.
“The shape of a molecule governs its function,” Li explains. “Think about it like locks and keys. If we can determine the shape of a lock — let’s say, a protein that helps tuberculosis bacteria survive — then we can develop a key, or medication, to interact with that lock. This is how we develop new, more effective tuberculosis drugs.”
Using cryo-EM, Li and his colleagues are searching for weaknesses in TB-causing bacteria. These vulnerabilities can be leveraged to design highly targeted — and more effective — treatments that could save lives and prevent transmission.
Their work comes at a critical time. Although advances in prevention and treatment have blunted tuberculosis’ impact, the disease still sickened more than 10 million people and claimed 1.3 million lives worldwide in 2022.3 More than 80% of these deaths were in low- and middle-income countries, where lack of resources and other social and systemic factors impede access to healthcare and lifesaving medicine.3,4 These challenges are compounded by the duration of tuberculosis treatment, which must be taken consistently for four to six months.3 Stopping treatment early can allow hardier TB bacteria to flourish, resulting in a resurgent infection that may resist frontline antibiotics.
When it comes to bacteria, “resistance” is a scary word. It means that the microbes that make us sick are no longer susceptible to the antibiotics we use to kill them. Some bacteria, including several strains of M. tuberculosis, have become resistant to multiple medications, resulting in a dangerous, tough-to-treat infection that stymies attempts to get it under control.
“Tuberculosis is treatable and curable,” Li says. “But drug-resistant strains are becoming more common. We need new strategies to fight back.”
Related: Learn more about VAI’s Cryo-EM Core ➔
Building a blueprint for better treatments
TB bacteria are tenacious. Like spies in the night, they infiltrate host cells, where they hide from the immune system. The bacteria then usually enter a latent stage, where they remain in host cells without causing illness or other symptoms. Roughly one quarter of the global population has latent tuberculosis; of those, 5%–10% will eventually become sick.3 Latent TB is not contagious.3
For scientists who study TB and the doctors and healthcare workers who treat it, the bacteria’s knack for hiding in our own cells is a big problem that complicates treatment. But it may not be able to hide for much longer.
Over the past several years, Li and his collaborators have visualized parts of the system that helps TB bacteria survive. The human immune system doesn’t make it easy — once in the body, the bacteria are bombarded by an immune firestorm, which damages enzymes required for their survival.5 As a result, TB bacteria have developed strategies to withstand the onslaught: repair salvageable enzymes and eliminate those that are irreparably damaged.
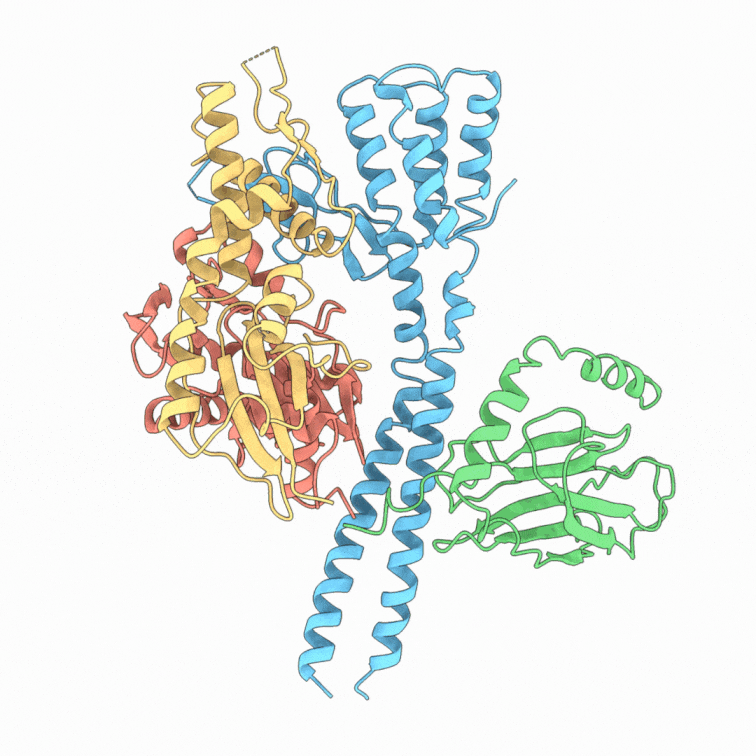
Using cryo-EM, Li and colleagues have visualized key parts of the system that rescues and repairs damaged enzymes. Called ClpB-DnaK, this system pulls apart damaged clumps of enzymes and ships the components to a molecular repair center, where they are reassembled (or refolded, in technical terms). 6 Most recently, in January, the team revealed how a specific part of the reassembly process, a molecule called GrpE, helps exchange materials required to rebuild damaged enzymes.7
“Repairing these broken enzymes is critical for survival of TB bacteria,” Li says. “We want to throw a wrench into the gears of this system — and one way to do that is by targeting these processes.”
Li and his colleagues also have their sights set on another system critical to TB bacteria. When the immune system damages bacterial enzymes beyond repair, TB bacteria fire up a trash removal system called Pup-proteosome. To date, Li and his colleagues have published more than 20 scientific studies detailing the innerworkings of Pup-proteosome and investigating compounds that interfere with its function while sparing similar systems in the human body.8
“TB bacteria need the Pup-proteosome system to survive,” Li explains. “Disabling the bacterial proteosome — while not interfering with human proteosomes — is another promising avenue for future treatments.”
This future may be closer than it appears. Along with collaborators at Weill Cornell Medicine, Li and his team have determined how several new inhibitors — compounds that stop a biological process — interfere with the bacterial proteosome.7 These insights, powered by cryo-EM, will help scientists optimize inhibitors to tackle TB.
Moving forward
Tuberculosis is a problem with a solution. Medications exist but, in too many cases, aren’t accessible to the people who need them. To fix this requires a concerted global effort to tackle systemic barriers. At the same time, we must develop new medications for multidrug-resistant strains of the bacteria.
For Li, hope for improved treatments is spurred on by cryo-EM and the breakthroughs it enables.
“Understanding the shape and function of molecules, like the enzymes that help TB infiltrate and survive in our cells, is the basis for more precise medications,” Li says. “Cryo-EM gives us unprecedented insights into the nuts and bolts of TB bacteria — and offers a chance to use this knowledge to fight back.”
Funding Acknowledgements
The research reported in Xiao et al. was supported by the National Institute of Allergy and Infectious Diseases of the National Institutes of Health under award nos. R01AI070285 (Li) and R01AI138446 (Glickman).
The research reported in Yin et al. was supported by the National Institute of Allergy and Infectious Diseases of the National Institutes of Health under award nos. R01AI070285 (Li) and U19AI111143 (Glickman); and the National Cancer Institute of the National Institutes of Health under award no. P30CA008748 (Glickman).
The research reported in Gang et al. was supported by the National Institute of Allergy and Infectious Diseases of the National Institutes of Health under award nos. P01AI056293 (Nathan) and R01AI070285 (Li); and the Milstein Program in Chemical Biology of Infectious Diseases.
The content is solely the responsibility of the authors and does not necessarily represent the official views of the National Institutes of Health or other funding agencies.
Sources
1 U.S. Centers for Disease Control and Prevention. (2023 October 18). History of World TB Day. www.cdc.gov/tb/worldtbday/history.htm
2 Rogers K. (n.d.). Tuberculosis through history. Brittanica. https://www.britannica.com/science/tuberculosis/Tuberculosis-through-history
3 World Health Organization. (2023 November 7). Tuberculosis. https://www.who.int/news-room/fact-sheets/detail/tuberculosis
4 Afranie Appiah M, Appiah Arthur J, Gborgblorvor D, Asampong E, Kye-Doudu G, Kamau EM, Dako-Gyeke P. 2023. Barriers to tuberculosis treatment adherence in high-burden tuberculosis settings in Ashanti region, Ghana: A qualitative study from patient’s perspective. BMC Public Health 23:1317.
5 Day NJ, Santucci P, Gutierrez MG. 2023. Host cell environments and antibiotic efficacy in tuberculosis. Trends Microbiol.
6 Yin Y, Feng X, Yu H, Fay A, Kovach A, Glickman MS, Li H. 2021. Structural basis for aggregate dissolution and refolding by the Mycobacterium tuberculosis ClpB-DnaK bi-chaperone system. Cell Rep 35(8):109166.
7 Xiao X, Fay A, Santos Molina P, Kovach A, Glickman MS, Li H. 2024. Structure of M. tuberculosis DnaK-GrpE complex reveals how key DnaK roles are controlled. Nat Commun 15:660.
8 Gang L, Li D, Sorio de Carvalho LP, Deng H, Tao H, Vogt G, Wu K, Schneider J, Chidawanyika T, Warren JD, Li H, Nathan C. 2009. Inhibitors selective for mycobacterial versus human proteasomes. Nature 461(7264):621–626.